New Satellite Sensors Continue to Improve Technique
In less than a decade, satellite-derived bathymetry (SDB) has evolved from a shallow-water mapping technology that could be applied only in clear, calm waters to one that can now be used cost-effectively and reliably in a wide variety of coastal environments around the globe. A 2023 project conducted in the challenging waters of Alaska was one of several that highlighted the arrival of SDB as a commercially viable, globally applicable seafloor mapping technique.
TCarta Marine of Denver, Colorado, successfully completed the Alaska project despite the presence of nearly every environmental condition that traditionally thwarted SDB production. The results were impressive: Seafloor depths were measured down to 26 m in Kachemak Bay and to 3 m in the murky and turbulent Arctic waters of Point Hope. Additionally, the data sets were delivered on time and within budget to the customer, NOAA’s Office of Coastal Management, and the data were made freely available to the public via the NOAA Digital Coast website: https://coast.noaa.gov/dataviewer/#/.
Fully appreciating the significance of this effort requires an understanding of SDB technology. It involves the analytical processing of the red, green, blue and infrared bands in optical multispectral satellite imagery. These spectral signatures captured by space-based sensors result from light energy penetrating the water column and reflecting off the seafloor. Processing these spectral reflectance values with specialized SDB algorithms calculates the depth of the water.
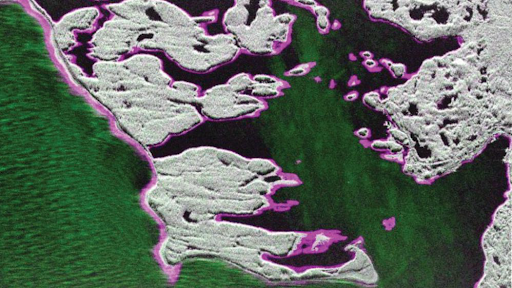
Numerous factors, however, can foil the SDB methodology. Darkness and cloud cover entirely prevent image collection by optical satellites, while the presence of sea ice and high levels of sediment or chlorophyll in coastal waters hinders light penetration, thereby eliminating seafloor reflectance and SDB derivation. The polar regions experience excessive cloudiness and six months or more without adequate sunlight for SDB. During summer months, glacial runoff creates highly turbid waters in addition to large-scale phytoplankton growth due to 24 hr. per day of light. Overall, the conditions for SDB in Alaskan waters are among the most challenging in the world. Despite these drawbacks, SDB is a popular mapping technique that has been applied reliably in many parts of the world and is a vital component to comprehensive coastal mapping programs. The primary benefit is that it can be used in shallow coastal zones that are too dangerous for shipborne methods, such as sonar, or too remote for deployment of airborne LiDAR.
Optical satellites, on the other hand, capture imagery globally across political boundaries and without risk to personnel, equipment, or the environment. The reduction in use of carbon-fuel-burning survey platforms is also a considerable benefit of satellite-based survey methods, and carbon costs are now becoming a consideration in international tenders for ocean mapping.
Geographic areas most amenable to SDB have traditionally included the Caribbean, Red Sea, Pacific atolls and the Arabian Gulf. Historically, SDB extraction from multispectral imagery was performed with one of two algorithms: Radiative Transfer or Band Ratio. The first is extremely computer intensive, sometimes taking days to process one image and requires extensive expertise. Band Ratio, on the other hand, is faster but requires calibration data, such as sonar or other direct depth measurement, which often aren’t available in remote or dynamic coastal zones, and high accuracy is limited due to areas of homogeneous seafloor.
The expansion of commercial SDB to parts of the world once thought impossible or impractical for it has resulted from a convergence of critical factors in just the past several years. These include dramatic improvements in satellite remote sensing capabilities, refinements in machine learning technologies to select images and derived water depths, and global availability of a secondary satellite-based bathymetric derivation method: space-based LiDAR.
The simultaneous emergence of these innovations has enhanced the quality and reliability of SDB measurements in places where it has always been applicable and expanded its technical viability to almost all environmental conditions and geographic locations. Just as importantly, the method can now be affordably applied in massive project areas, even if time and budgets are limited.
This is just the start of a paradigm shift in SDB methodology and applicability. There are additional advancements now being researched—mainly in the form of new satellite imaging technology—that will continue to enhance satellite-derived bathymetry.
Mapping the Alaskan Coastline
The success of the Alaska project would likely not have been possible if it weren’t for the research and development conducted under two Small Business Innovation Research (SBIR) grants awarded to TCarta by the National Science Foundation and NOAA between 2018 and 2022 with the goal of modernizing SDB techniques and expanding the pool of usable sensors. The research focused on overhauling the entire SDB workflow, devising tweaks to the algorithms themselves, as well as experimenting with new and better data imagery selection, data cleaning techniques and accuracy assessment.
The NOAA grant sought to specifically improve SDB outcomes in high-latitude areas such as Alaska and the Arctic. TCarta worked on the theory that notoriously turbulent coastal zones in this region experienced some calm days suitable for SDB. But pinpointing such ideal conditions that also coincided with periods of daylight dramatically narrowed the potential acquisition window of optical satellite image collection.
Fortunately, the availability of SDB-suitable imagery has exploded over the past 25 years with the launches of numerous optical imaging constellations. Gone are the days when the U.S. Landsat and French SPOT satellites were the only image sources, with imagery collected once a week at best. Today, there are numerous Earth observation systems in orbit operated by Maxar, Airbus, European Space Agency (ESA), Pixxel, Planet, Satellogic, and several others that provide a plethora of images to select from.
These satellites have been capturing imagery for years and amassing enormous archives of data covering the entire Earth. In many cases, these archives contain deep stacks of numerous images for each spot on the globe, some with hundreds of images to select from. In addition to more frequent acquisitions, some of these sensors capture reflectance data in different segments of the optical and infrared spectra, which enhance SDB by penetrating deeper into the water column, providing additional seafloor information and enabling more robust corrections for atmospheric distortions.
TCarta had been modifying the original SDB derivation algorithms with the addition of these new spectral bands and ratios of multiple bands and then applying machine learning to recognize the best results from the seafloor extraction process. It was a natural step to then apply machine learning to stacks of archived satellite images and find the ones with the clearest, calmest water conditions for SDB processing. From there, the process was refined to use machine learning to isolate and combine the best pixels from multiple overlapping images for derivation of water depths. Searching the archives manually to find images with the right characteristics would be impossible or too time consuming for practical application. Machine learning makes this possible.
For Alaska, TCarta processed high-resolution multispectral imagery from the Maxar and Planet archives to complete the project. These data sets were chosen for their high spatial resolution and frequency of imagery collection, which were required to meet the bathymetric accuracy requested by NOAA’s Office of Coastal Management. TCarta used a custom process to assimilate the radiometric measurements of the two different sensor systems for consistent SDB extraction.
Incorporation of machine learning into the SDB processing workflow has substantially reduced production time. TCarta routinely processes dozens to hundreds of images in the same time it would have taken for one just a few years ago. This has substantially improved the overall economics and viability of SDB as a commercial service.
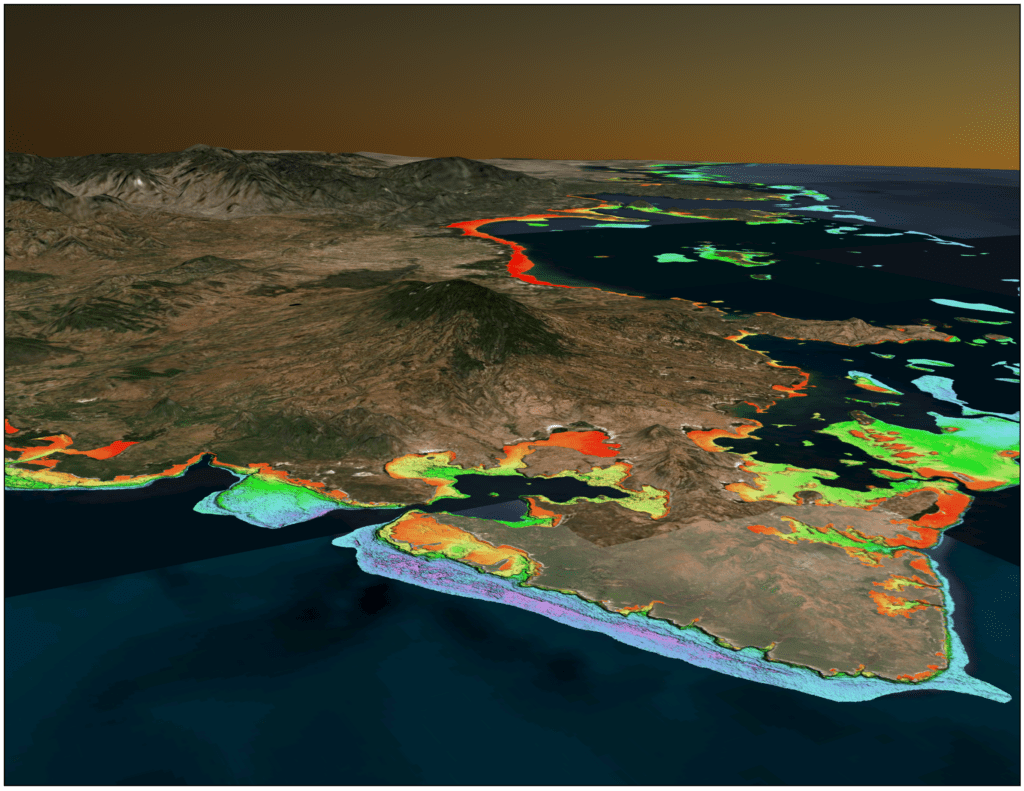
Adding Satellite LiDAR for Validation
The other major addition to the SDB workflow has been space-based LiDAR, or laser altimeter, data from NASA’s ICESat-2 satellite. Launched with the intent of measuring the thickness of sea ice, glaciers and tree canopies, the satellite also directly measures seafloor depth down to about 30 m under the right conditions. The LiDAR’s green laser emissions penetrate shallow water, reflect off the bottom, and return a signal to the sensor. The data can be processed to determine depth to high levels of accuracy.
The ICESat-2 measurements are made in single-point survey measurements with approximately 1 m between points along the track and with several kilometers between each track, which means the data cannot be used as a standalone bathymetric mapping tool for broad areas, but its value is still considerable.
ICESat-2 has proved to be the ideal validation and calibration data set that extends SDB utility to remote parts of the world where no control points can be collected or are otherwise unavailable from on-site methods. The ICESat-2 data are applied in two aspects of the modernized SDB workflow. The laser measurements are first employed as training data to train the machine learning algorithms to recognize water depths in optical imagery. The ICESat-2 points are also used to validate the accuracy of SDB calculations derived from the Radiative Transfer SDB method, enhancing the confidence in derived water depth values. By combining both methods and information from two satellites using independent methods for water depth derivation, greater confidence in the results is achieved.
Although TCarta uses the NASA data in all SDB projects now, the value of the data set was demonstrated most vividly in another 2023 project where TCarta performed bathymetric mapping of the entire Madagascar coastline. Mapping the coastal zone of the fourth largest island on Earth was noteworthy for several reasons, aside from its sheer size and remoteness. First, the project was supported by the Seabed 2030 program, which was also the recipient of the final products. Second, TCarta completed the SDB mapping in cooperation with students during a summer work term program at the Marine Institute (MI) of Memorial University in St. John’s, Newfoundland, Canada.
Due to a limited budget and grand ambitions to make large contributions to Seabed 2030, free imagery from the 10-m-resolution ESA Sentinel-2 satellite was used. Thousands of satellite images, many stacked over the same areas of interest, were obtained for the entire Madagascar coast. Without machine learning and ICESat-2, the SDB mapping project would have taken 12 months or more just a few years ago, but the student-involved teams completed it in a few weeks, achieving 24-m-deep measurements along the majority of the Madagascar coastline.
The technology and techniques developed under TCarta’s research grants have not only gone on to benefit the commercial and government projects, but also it has proven to be a tremendous instructional tool for future hydrographers and contributed significant data coverage to the global effort to map the entirety of the seafloor.
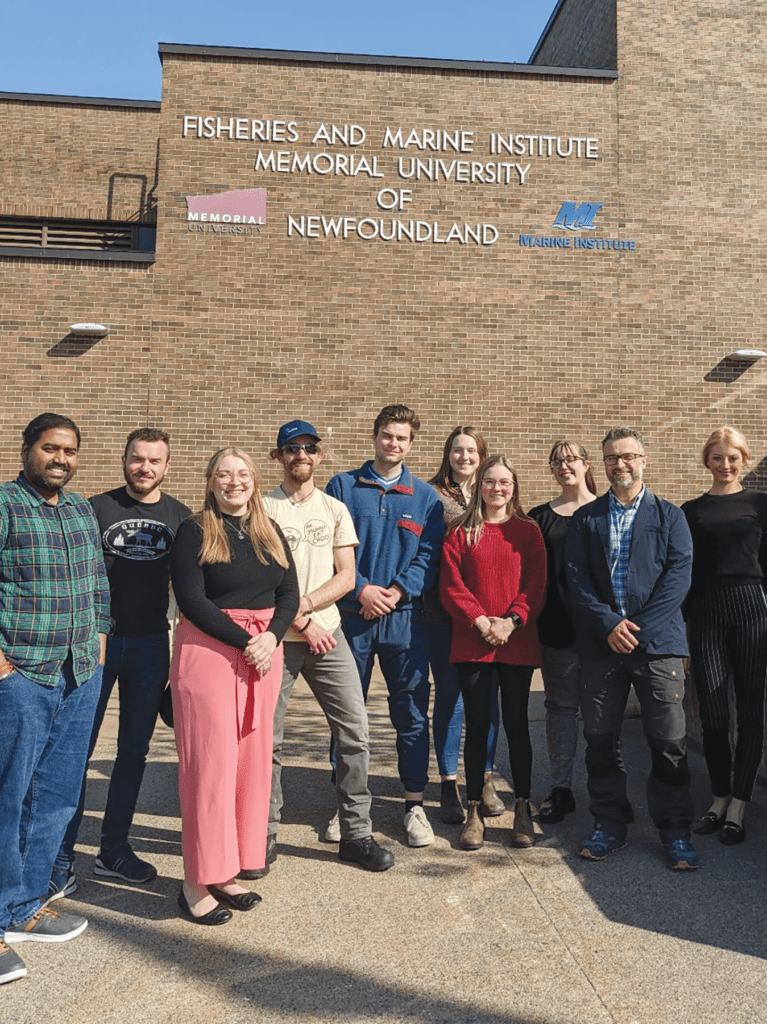
The Future of SDB
The revolution in commercial imaging satellites has included two types of data collection systems that hold significant potential for SDB applications and supplemental coastal products. These are synthetic aperture radar (SAR) and hyperspectral satellite constellations. The primary advantage to SAR is that, unlike passive optical systems that capture reflected sunlight, radar sensors actively emit signals that can pass through darkness and clouds to bounce off the Earth’s surface and return data. This means they can collect data 24/7 anywhere on the globe. The key difference in recent SAR missions is their spatial resolution supports practical coastal mapping. Commercial SAR operators include Capella Space and Umbra in the U.S., ICEYE of Finland, and Synspective of Japan.
While SAR does not provide seafloor depth information due to lack of water column penetration, it does add important shoreline location data that can enhance SDB accuracy. TCarta has used the radar data collected at both day and night in some high-latitude projects to precisely map high- and low-tide water levels to determine the extent and characterization of the intertidal area. These shoreline features can be applied as a complement to the SDB products, but it is often requested by some commercial clients as a standalone coastal map product.
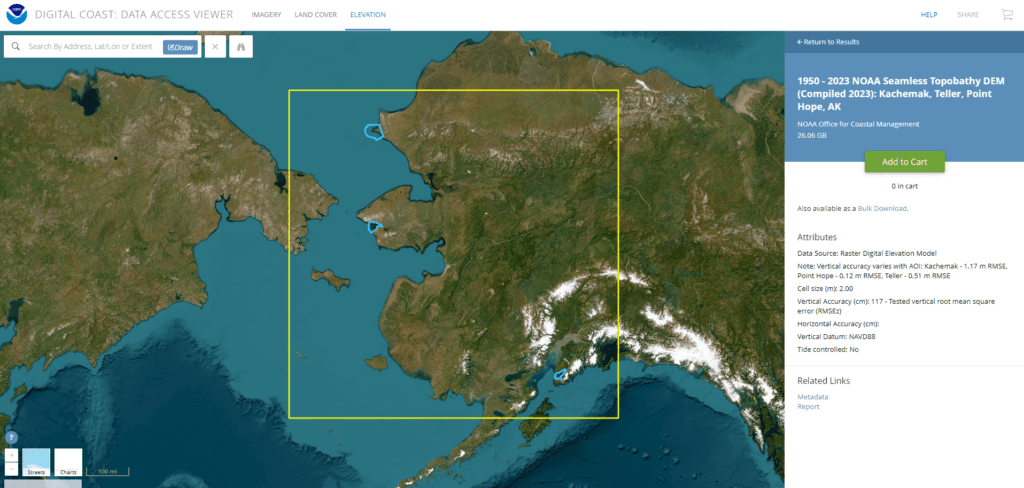
TCarta has teamed with Capella on joint research projects to determine other ways high-resolution SAR can be incorporated into marine mapping and possibly SDB projects. As an analytic partner, TCarta has been developing coastal products and tools for ready use by customers around the globe. The potential for hyperspectral satellite data to directly impact SDB by facilitating deeper and more accurate seafloor measurements is even more significant. Generally referring to sensors that acquire reflected data in more than 10 spectral bands, hyperspectral systems have recently been launched by several companies: Pixxel in India, Wyvern in Canada, Orbital Sidekick of the U.S. and others.
The excitement for SDB analysis is that these sensors capture reflected energy in very narrow bands across the visible and infrared portion of the spectrum. Initial research by TCarta in cooperation with Pixxel indicates these narrow bands, especially in visible green and blue, will detect reflected energy that has passed more cleanly through the atmosphere without distortion and penetrate deeper into the water column. This will potentially extend the useful range of SDB into deeper, and possibly less clear, water.
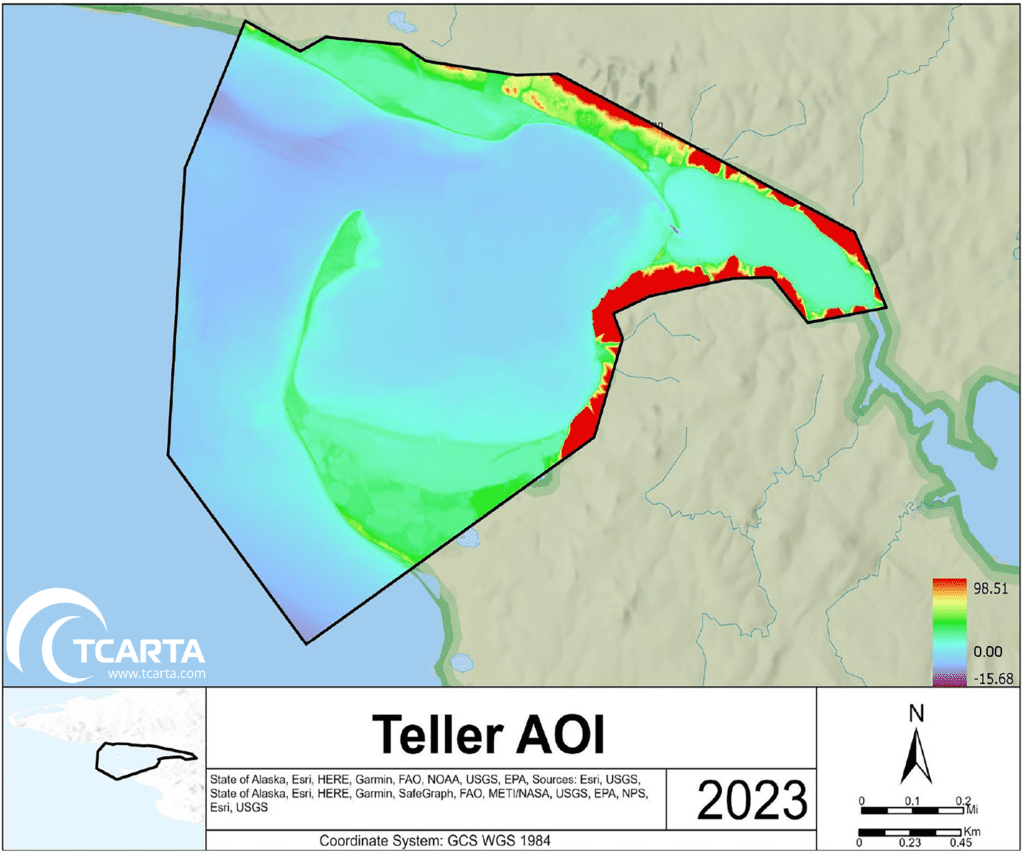
While more advanced satellite imaging platforms will continue to play key roles in SDB progress, the SBIR grants from the U.S. government have most directly impacted shallow-water mapping capabilities for the hydrographic and hydrospatial communities. These investments are paying off in terms of safer coastal navigation, more responsible shoreline development, and more diligent environmental protection in the littoral zone.
Kyle Goodrich is the president and founder of TCarta Marine and has a 22-year career in geospatial services. Since founding TCarta in 2008, Kyle has led numerous geospatial product research and development plans, including the development and commercialization of satellite-derived bathymetry, stereo photogrammetric bathymetry, a global aggregation and assimilation of multi-source bathymetry data and global vector shoreline. As principal investigator in TCarta’s National Science Foundation and NOAA Small Business Innovation Research Phase Two projects and as an active industry member in international hydrographic commissions, Goodrich is a persistent and passionate leader in the commercialization of satellite-based marine remote sensing technologies.
You can also view this article on TCarta’s LinkedIn: https://www.linkedin.com/pulse/global-satellite-derived-bathymetry-tcarta-marine-ufmvc/?trackingId=ClUjZSlmjUmSmhTxUKXY3A%3D%3D