Accurate and timely environmental data is critical for the success of hydrographic surveys. One increasingly valuable tool in this domain is space-based water quality monitoring, which offers powerful support for airborne LiDAR bathymetry programs. By assessing daily and historical water clarity conditions from satellite imagery, this method provides essential guidance for flight planning, acquisition timing, data quality assurance and reporting.
Why Water Quality Matters for LiDAR
The performance of bathymetric LiDAR systems is highly dependent on water clarity. Turbid or sediment-rich waters scatter and absorb the laser pulses used in LiDAR surveys, reducing their ability to penetrate the water column and reach the seafloor. This makes understanding water quality—especially parameters like turbidity, suspended particulate concentration, and light attenuation—vital for ensuring successful, cost-efficient LiDAR acquisitions.
Existing methods rely on in situ measurements, which are costly and require mobilizing personnel and equipment. As a result, data collection is often limited to a few points. Alternatively, some approaches depend on local knowledge or visual assessments from the air. While these approaches can help determine broad acquisition windows, they often lack spatial and temporal precision which is vital for detailed planning and contextualizing data quality.
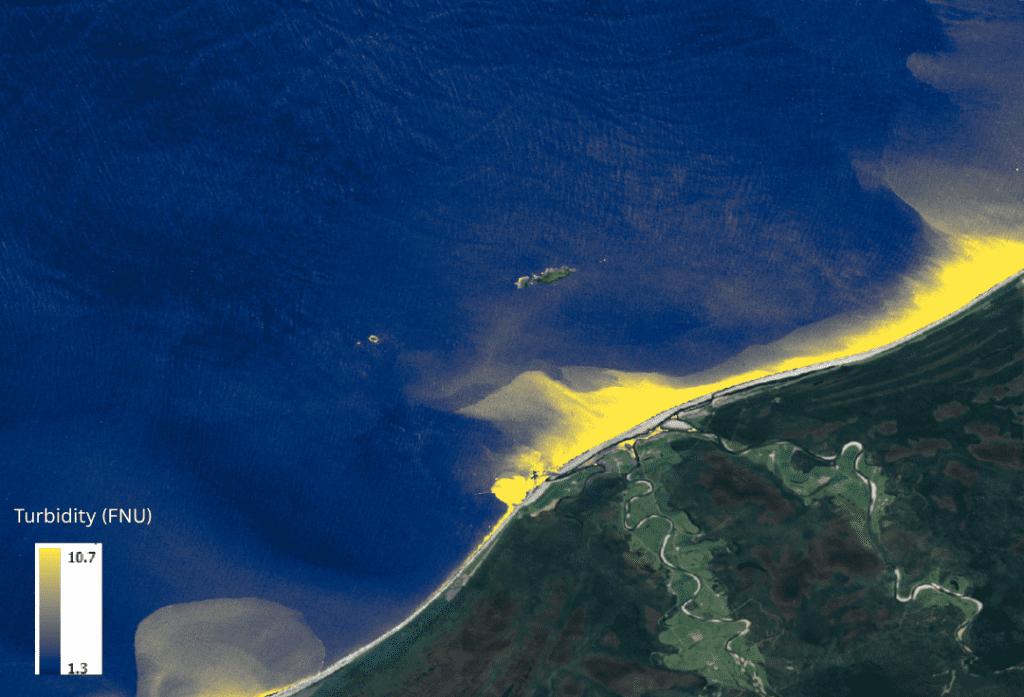
Space-Based Monitoring: A Strategic Advantage
Using high and moderate resolution multispectral satellite imagery from platforms such as Sentinel-2 and PlanetScope, TCarta offers a robust and cost-effective satellite water quality monitoring service. This service enables both historical and near daily evaluation of water conditions across survey sites.
For planning bathymetric lidar flights, satellite-derived data can:
- Identify optimal collection windows when water clarity is most favorable.
- Detect turbidity plumes that may limit LiDAR acquisition.
- Support adaptive flight planning, adjusting flight plans or survey sites to maximize success based on physics-based analysis.
- Contextualize data quality after acquisition.
Core Water Quality Metrics
Key parameters analyzed through satellite-based methods include:
- Kd490: The diffuse attenuation coefficient at 490nm (blue/green). Quantifies the rate at which blue/green light is absorbed and scattered as it travels through the water column.
- bb490: The backscattering coefficient at 490nm. Indicates the amount of blue/green light scattered back towards the sensor by particles and other constituents in the water column.
- bbp533: Particulate backscattering coefficient at 533nm. Indicates the amount of green light scattered back toward the sensor by particles. A good proxy for LiDAR laser penetration.
- Secchi Depth: An indication of how deep light can penetrate into the water column using a simulated Secchi disk.
- SPM: An estimate of the amount of suspended particulate matter in the water column.
- Turbidity: The amount of scattered light proportional to the concentration of particles in the water. Sensitive to particle size, shape, composition, density, and color.
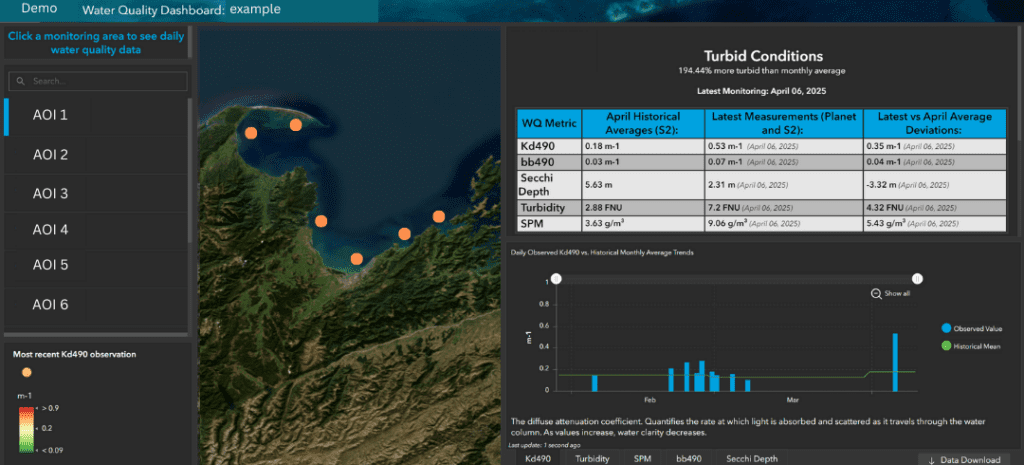
By combining daily PlanetScope observations with historical Sentinel-2 data, daily monitoring data can be evaluated against historical trends for more accurate anomaly detection. This customizable multi-temporal approach helps surveyors understand both seasonal patterns and real-time shifts in water clarity, supporting strategic mission execution.
Broader Applications: From Planning to Environmental Monitoring
In addition to supporting LiDAR surveys, TCarta’s water quality monitoring is also used across multiple sectors. One key application is the detection and tracking of harmful algal blooms (HABs), which pose risks to ecosystems, public health, and fisheries. Industries such as aquaculture, coastal engineering, and marine construction also benefit from reliable, up-to-date water quality information.
Deliverables
Clients receive access to a customized and dynamic web dashboard along with downloadable reports, graphs, and time series charts. These tools allow users to:
- Monitor trends over time.
- Compare current water conditions with historical baselines.
- Make informed decisions during and after survey operations.
- Report on water conditions as they relate to collection success.
- Identify if reflights to infill data gaps will be successful.
Case Study: Florida Coastal Mapping:
A Smarter Approach to Survey Success
Space-based water quality monitoring transforms how bathymetric LiDAR missions are planned and executed. By giving surveyors “eyes in the sky,” it enhances operational efficiency, data quality, and overall mission outcomes.
As a leader in satellite-derived marine data solutions, TCarta offers satellite-based Water Quality Monitoring as a key service for LiDAR survey planning and operational support. This forward-looking approach continues to revolutionize the way we collect, analyze, and act on hydrospatial information.